Wednesday, September 30, 2020
Lupine Publishers: Lupine Publishers | Information and Communication ...
Tuesday, September 29, 2020
Lupine Publishers: Lupine Publishers | Allele Mining for the Reported...
Monday, September 28, 2020
Lupine Publishers: Lupine Publishers | Lipoma of the Parotid Gland: A...
Friday, September 25, 2020
Lupine Publishers: Lupine Publishers | Evaluation of Combinatorial Ca...
Thursday, September 24, 2020
Lupine Publishers: Lupine Publishers | Intervarietal Hybridization an...
Wednesday, September 23, 2020
Lupine Publishers: Lupine Publishers | Perceived Effects of Resource-...
Friday, September 18, 2020
Lupine Publishers: Lupine Pubishers | Dimethyl ether as Zero Emission...
Thursday, September 17, 2020
Lupine Publishers: Lupine Publishers | Biogas and Dimethyl Ether are ...
Wednesday, September 16, 2020
Lupine Publishers: Lupine Publishers | Biogas and Dimethyl Ether are ...
Tuesday, September 15, 2020
Lupine Publishers: Lupine Publishers | Statistical Software: A Risk f...
Monday, September 14, 2020
Lupine Publishers: Lupine Publishers | Management of Internal Root Re...
Friday, September 11, 2020
Lupine Publishers: Lupine Publishers | About the Influence of Evapora...
Thursday, September 10, 2020
Lupine Publishers: Lupine Publishers | Fresh Water-an Emerging Global...
Lupine Publishers| Diabetes Mellitus: Classification, Genetic Association and Herbal Treatment
Lupine Publishers| Diabetes Mellitus: Classification, Genetic Association and Herbal Treatment
Abstract
Diabetes Mellitus (DM; chronic metabolic disorder) is turning out to be pandemic with India (2nd most populous country) as its epicenter; preying on health and economy of people of all ages, sex, and ethnicity. It affects all the major systems of the human body and is an underlying factor for all the other ailments, including cancers. Available anti-diabetic drugs are only able to reduce the detrimental effects of diabetes and its related complications, but still, the complete cure is unabated. Additionally, in low-middle-income countries, the economic burden of drugs on the common man is a major hindering issue in the management of disease outcome. Among the general population, the most commonly known types of this disease are type I and type II DM. Despite strenuous efforts taken by governments throughout the world, including India; due to asymptomatic nature and lack of awareness, there is still lag in understanding and management of other less commonly known DM’s. This review highlights the detailed classification of DM’s and the underlying genetic factors. As well as enlist few cost-effective herbal therapies to combat the disease.
Keywords:Diabetes Mellitus; Gestational Diabetes; Classification; Diabetic Ketoacidosis; Genes; Herbal Therapy; Diabetic complications
Abbreviations:DM: Diabetes Mellitus; GDM: Gestational Diabetes Mellitus; DKA: Diabetic Ketoacidosis; MODY: Maturity Onset Diabetes of the Young; FCPD: Fibrocalculous Pancreatic Diabetes; MIDD: Maternally Inherited Diabetes with Deafness; RAAS: Renin Angiotensin Aldosterone System; eNOS: Endothelial Nitric Oxide Synthase
Introduction
Diabetes mellitus (DM), a disease of the endocrine pancreas in which either pancreatic islets secrete insufficient insulin, or there is inefficient utilization of insulin; thus, producing hyperglycemic effects and other metabolic complications. According to the global estimates of American Diabetes Association; 500 million (age range 18-99 years) were diabetics in 2018 and the number could escalate to 693 million by 2045 (International diabetes federation; 2017). Further, half of the total diabetics were asymptomatic and undiagnosed [1]. Hence, DM and its associated complications are becoming one of the major causes of mortality [2]. The serious detrimental stride of diabetes is that it could enhance the risk of developing cancers in various organs such as liver-, breast-, bladder-, kidney- and bladder [3]. The risk factors contributing to diabetes include- genetics, environment, lifestyle, and certain drugs [4]. Unlike in the past centuries; the disease is currently becoming pandemic - affecting all ages and sex and its management including economic and social burden is of concern for policy makers of high-, middle- and low-income countries [3].
DM Classifications
In addition to well-known type –I and – II DM; pregnancyassociated gestational DM (GDM) is the third most common DM. Other types include Monogenic DM (MODY), Fibrocalculous Pancreatic DM (FCPD) and Maternally Inherited Diabetes with Deafness (MIDD). Another variant of this complex disease includes genetic syndrome, certain drugs and diseased conditions, infections and hormonal- induced DM [5,6]. Brief descriptions about different types of DMs’ are as follows.
Type I DM: Type-I or insulin-dependent DM; is an autoimmune condition in which the pancreatic islets are destroyed by the cytotoxic and delayed hypersensitive T lymphocytes. The disease susceptibility increases in HLA – D type inherits, especially in children and young adults; hence classified as Juvenile - onset diabetes; it accounts for 5-10% of all people with diabetes. As proposed by the WHO and American Diabetics Association, type I diabetes can be classified as type IA and type IB. Type IA is immunemediated diabetes destroying pancreatic β cell and absolute insulin deficiency. Type IB is idiopathic diabetes in which the diabetics lack the immunological marker for autoimmune destruction of the pancreatic β cell. This form of DM also results in insulin deficiency and a tendency to develop ketosis, thereby requiring exogenous insulin as a therapeutic agent [3].
Diabetic Ketoacidosis (DKA): DKA is an acute life-threatening complication characterized by hyperglycemia (>250 mg/dl), metabolic acidosis (pH ˂ 7.3) and serum bicarbonate (˂ 15 mEq/ dl) with a significantly raised anion gap and presence of ketone bodies in the blood and/or urine [7]. Inadequacy of insulin dosing/ schedule, non-compliance or infrequent insulin injection and infection are major factors for its inception [7]. In 44% of DKA cases, non-compliance is the causative factor for its onset. Ailments of cardiovascular systems including myocardial infarction, stroke, and acute thrombosis; gastrointestinal tract disorders such as bleeding and pancreatitis; endocrine-related diseases such as acromegaly, Cushing`s syndrome and hyperthyroidism are some other contributors for the onset of DKA. In certain instances, even recent surgical procedures leads to the development of DKA caused mainly due to dehydration, increased insulin counter regulatory hormones, and worsening of peripheral insulin resistance [8]. Additionally, even a class of type II management drug - sodiumglucose cotransporter -2 (SGLT-2) inhibitors were reported to induce DKA; other classes of drugs such as, beta-blockers, corticosteroids, second-generation anti-psychotics, anti-convulsants and immune checkpoint inhibitors adds to the list. Non-drug-induced DKA includes psychological problems, binge eating and insulin pump malfunction.
Pathophysiology of DKA: In DKA, complete lack of insulin leads to impaired glucose utilization, which in-turn results in gluconeogenesis, glycogenolysis and decreased peripheral glucose uptake and thereby leading to hyperglycemia. In addition, impaired glucose utilization also leads to an increase in lipolysis, decreased lipogenesis and overproduction and urinary loss of ketone bodies [7]. In addition, glycogen and protein is catabolised as an alternative energy source to form glucose. Combination of these factors contributes to the formation of hyperglycemic condition, leading to osmotic diuresis which ultimately results in dehydration, metabolic acidosis and hyperosmolar state and subsequent decrease in glomerular filtration [8]. Potassium utilization by the skeletal muscles is highly depleted due to impaired insulin action and hyperosmolar state, resulting in intracellular potassium depletion [8].
Type II DM
Type II DM is the most common and accounts for 90-95% of all diabetes types [3]. This type of diabetes also called as non-insulin dependent DM and there is no exogenous dependence on insulin by the type II diabetics, but it may be required in case of uncontrolled blood glucose levels [3]. Interaction between genetics, environment and behavioral risk factors leads to the progression of type II DM [9].
Pathophysiology: Type II DM is characterized by hyperglycemia, insulin -deficiency and -resistance and eventually β-cell failure [9]. Insulin resistance is a condition in which there is impaired insulin action in proportion to its blood concentration. Sufficient amount of insulin is secreted by the pancreatic islets, but the tissues are relatively insensitive to insulin action and show inadequate response towards it. β-cells maintain normal glucose tolerance by increasing the insulin output. When β-cells are not able to release sufficient insulin for glucose uptake leads to elevation in glucose concentration. The threshold at which glucose stimulate insulin secretion is determined by glucokinase, which also mediates the conversion of glucose to glucose- 6-phosphates. In insulin resistance, pancreatic β-cell is marked with increased hexokinase activity, rendering the secretion of insulin at lower glucose concentration. It has been suggested that β-cell failure could be induced by apoptosis on long-term exposure of pancreatic islets to free fatty acids which inhibits glucose-induced insulin secretion and biosynthesis [3].
Insulin Action: Insulin-induced effects are seen through modulation of cytosolic enzymes and the control of gene expression. The general action of insulin is to promote anabolic process in a cell, while causing a reduction in catabolic process. Insulin is detected on the plasma membrane of the target cell by insulin receptor (INSR), which is a tetrameric glycoprotein contains insulin-binding two α subunits, and tyrosine kinase-binding two β subunits [10]. Insulin binding to INSR leads to its activation and involve signaling events which can be functionally divided into mitogenic and metabolic signals [10].
a) Mitogenic Signals
Mitogenic signals involve activation of mitogen-activated protein kinase (MAPK) pathway, which is activated when IRS interacts with adaptor protein Grb2 through its SH2 domain. Grb2 also binds to Son of Sevenless (SOS), which is a guanine nucleotide exchange factor (GEF) and catalyses the exchange of GDP for GTP on Ras, which in turn activates Raf kinase and lead to the phosphorylation and activation of MAP kinase cascade [11]. One of the end results of the activation of this cascade is the alteration of gene expression. MAPK pathway may regulate DNA binding, protein stability, cellular localization, transcription, repression, and nucleosome structure by targeting components at gene promoters. These pathways alter gene expression through phosphorylation and regulation of transcription factors, co-regulatory proteins and chromatin proteins [12].
b) Metabolic Response
Metabolic activities of insulin primarily involves catabolic (action of glycogen phosphorylase), anabolic (action of glycogen synthase) and PI3K pathway mediated GLUT4 translocation. Activated INSR phosphorylates protein phosphatase 1 (PP1), which dephosphorylate glycogen synthase (activating it) and glycogen phosphatase (inactivating it), leading to increased glycogen synthesis and inhibition of glycogenolysis. Activated INSR also leads to the activation of PKB. Glycogen synthase kinase 3β (GSK3β) is one of the substrates of PKB, which becomes inactivated upon phosphorylation and leading to inhibition of glycogen synthase activity [11]. Multi-phosphorylation of insulin receptor substrate (IRS), essentially IRS1 and IRS2 marks the key event in the signal transduction. On phosphorylation, IRS1 creates binding sites for the SH2 domain of other proteins such as PI3K. Activated PI3K leads to the production of PIP2 and PIP3, which in turn activate phospho-inositide dependent kinase 1 (PDK1). PDK1 is capable of phosphorylation and activation of PKB and PKC. Signaling events from PKB and PKC will lead to the translocation of GLUT-4 transporter to the plasma membrane (Figure 1).
Gestational Diabetes Mellitus (GDM)
GDM is a well- known variant of DM that develops during pregnancy and ceases at its end [11]. GDM can be defined as any degree of glucose intolerance which is detected for the first time during the gestational period. Women with marked obesity, glycosuria, personal history of GDM or family history of diabetes are at high risk of developing GDM. The diagnosis criteria for GDM include a fasting plasma glucose >126 mg/dl (6.9mmol/l) plus a 2-h value of 140-199 mg/dl (2.9- 11.1 mmol/l) or casual plasma glucose > 200mg/dl [13]. Due to high levels of placental hormone and with an increase in maternal adiposity, there is a progressive increase in insulin resistance and a decrease in insulin sensitivity. These changes are accompanied by changes in the glucose levels which reverse upon delivery in normal condition. Women who are not able to respond appropriately to these pregnancies related changes become hyperglycemic and develop a condition called GDM [14]. Pregnancy-related complications like diabetic fetopathy, fetal macrosomia, still births, neonatal metabolic disturbance, etc. are associated with gestational diabetes. Problems exhibited by hyperinsulinemic infants include hypocalcemia, hyperbilirubinemia and plethora. Women who had developed GDM are at seven fold higher risk of developing diabetes in later years [5]. The risk of getting diabetes and obesity increases in children born with gestational diabetic mothers [13].
Other Specific Types of Diabetes
Maturity-Onset Diabetes of the Young (MODY): MODY is a heterogeneous form of DM that is caused by a single gene defect. MODY is non-ketonic diabetes characterized with an autosomal dominant mode of inheritance, partly preserved pancreatic β-cell function and an early onset usually before 25 years of age [3, 15, 16]. MODY 1-14 types have been reported [17]. MODI 1 is caused by a mutation in HNF4A gene while a mutation in GCK gene is responsible for MODY 2 and is characterized by the occurrence of phenotypes immediately after birth and a mild increase in fasting glucose level [18]. The gene for MODY3 is HNF1A [19] and its clinical features includes hyperinsulinemic hypoglycemia in the neonatal period and macrosomia [18]. The onset age of MODY 3 is dependent upon the paternal or maternal inheritance of mutation and also on the maternal affected status when a mutation is of maternal inheritance [15]. Mutation in exon 4 of HNF1A results in the generation of pancreatic autoantibodies and diabetic ketoacidosis [19]. However, MODY 4 has not yet been reported in India and Japan [5,15]. MODY 5 is caused by the defects in transcription factor - hepatocyte nuclear factor 1 homeobox B (HNF1B). In MODY 5 insulin secretion is decreased since the early stage but is often diagnosed during adulthood. This form is characterized by frequent mutations of monoallelic defects in all or some exons and by multiple phenotypes most commonly being renal diseases. MODY 6 is caused by the mutation in the transcription factor NEUROIDI, which is known to be involved in gene activation of sulfonylurea factor 1 and expression of GCK gene thus contributing to different roles from synthesis to secretion. Homozygous mutation in NEUROIDI gene leads to neurological abnormalities and permanent neonatal DM [20].
Fibrocalculous Pancreatic Diabetes (FCPD): FCPD is an unusual diabetes phenotype restricted exclusively to the developing countries residing in the tropical regions of the world with the highest prevalence in southern India [21,22]. FCPD is predominantly described in lean young adults of either sex with a long- standing history of abdominal pain and steatorrhea. FCPD is non-alcoholic chronic pancreatitis that results from a specific type of pre-existing diabetes termed as Tropical Calcific Pancreatitis (TCP) [23]. Severe insulin dependent but ketosis resistant diabetes occurs in FCPD and patients with this phenotype develop microvascular complications and are more prone to develop dreaded complications like pancreatic malignancy [21, 24]. Although the exact etiology of FCPD has not yet been elucidated, it has been proposed to be associated with malnutrition, cassava consumption (cyanogens toxicity), toxins, micronutrient deficiency, oxidative stress, anti-oxidant depletion, free radical injury, beta cell autoimmunity and genetic factors [22,25]. HLA-DQ9 allele, HLADQB1 allele, loss of function mutation in chymotrypsinogen C gene and cathepsin B gene, SPINK1 gene (serine protease inhibitor), and CFTR gene are some of the genetic factors involved in the pathogenesis of FCPD [22].
Maternally Inherited Diabetes with Deafness (MIDD): MIDD, caused by mitochondrial gene mutations, is a rare form of diabetes characterized by pancreatic disorder and hearing impairment [26]. It has been reported that MIDD not only affect two organs i.e. pancreas and ears leading to diabetes, hypoacusis and anacusis; but has been found to manifest its effects on multiple organs and hence termed as mitochondrial multi-organ disorder syndrome (MIMODS) [27]. MIDD could confer its effect on the central nervous system (CNS), endocrine organs, peripheral nerves, ears, eyes, kidneys, heart, vestibular system and gastrointestinal tract [27]. Subjects affected with MIDD are under high prevalence of developing microvascular and macrovascular complications and rapid progression to insulin therapy [28].
Secondary Causes of Diabetes: Exogenous factors and some specific disease condition could also secondarily develop diabetes. For example, intake of some medications like corticosteroids, calcineurin inhibitors, thiazide diuretics, antipsychotics, HIV protease inhibitors, γ-interferon and statins may lead to the development of diabetes [26]. Drugs like nicotinic acid and glucocorticoids can affect to impair glucose action. Viral infections like hepatitis C infection, rubella, mumps, coxasckievirus, cytomegalovirus and adenovirus have been associated with β- cell destruction [29]. Hormonal excess, including growth hormone, cortisol, glucagon, epinephrine are some other secondary causes of diabetes. Genetic syndrome like Down syndrome, klinefelter syndrome, Turner syndrome, Wolfram syndrome, Huntington chorea, porphyria, etc. are accompanied by an increased incidence of diabetes. Specific disease conditions like stiff-man syndrome, systemic lupus erythematosus and other autoimmune diseases have anti-insulin receptor antibodies that block insulin binding to its receptor [26] Figure 2.
Genes Associated with DM
Type I DM
Type I diabetes is a multifactorial disease with a strong genetic association. Family history association for the disease is missing in about 85% cases with TI DM; however, evidence suggests that the risk of developing TI DM is 15 times greater in siblings of the diseased than in the normal subjects [30]. Moreover, the concordance rates for TI DM in monozygous twins are high upto 33% to 40% [31].
HLA Alleles: HLA class II allele present on chromosome 6p21 with loci HLA-DRB1 and HLA-DQB, accounts for up to 30% -50% of genetic risk for TI DM. The haplotypes DR3-DQA1*0501- DQB1*0201 (DR3) and DR4-DQA1*0301- DQB1*0302 (DR4) are at the greatest risk for T1D (32). HLA-A, - B & -C alleles have also been known to be associated with T1D independent of DR/DQ [33,34].
Non- MHC Genetic Factors: Multiple non-MHC loci including insulin, PTPN22, CTLA4, IL2RA, IFIH1, etc have been confirmed as susceptible gene markers for TI DM. It has been reported that after HLA alleles, the genetic contribution of INS gene and PTPN22 genes have been the most with reference to the diabetes risk, however, even the strongest non-MHC loci have smaller effects [33].
Type II DM
There is a strong inheritable genetic correlation in type II diabetes. Studies have found that there is 40% lifetime risk of developing TII DM for the offspring who have one of the affected parents and the risk increases upto 70% for the offspring with both the affected parents [35]. Moreover, the concordance rate of about 70% has been observed in monozygotic twins and about 20%- 30% in dizygotic twins [36]. Multiple genes involved in insulin signaling, insulin secretion, insulin resistance, glucose metabolism, obesity, diabetes co-morbidity and hormone processing protease genes have been known to be associated with TII DM [37]. Unlike TI DM, multiple gene interactions results in the progression of TII DM. A number of TII DM risk genes have been identified using candidate gene studies, linkage-based studies and genome-wide association studies (GWAS) including transcription factor 7-like 2 (TCF7L2), calpain 10 (CAPN10), peroxisome proliferator-activated receptor gamma (PPARG), insulin receptor substrate 1(IRS1), IRS2, potassium inwardly–rectifying channel, subfamily J (KCNJ11), Wolfram syndrome 1 (WFS1), HNF1 homeobox A (HNF1A), HNF1B, HNF4, hematopoietically expressed homeobox (HHEX), insulin-like growth factor 2 mRNA binding protein 2 (IGF2BP2), FTO, NOTCH2, JAZF1, SLC30A8 and others [9,29]. More than 40 genetic loci have been known to be linked to TII DM. Some of the recently discovered genes that may have a profound impact on the progression and pathogenesis of TII DM are mentioned below.
Renin Angiotensin Aldosterone System (RAAS): The RAAS is considered as an endocrine system which consists of several RAAS genes including renin, angiotensinogen (AGT), angiotensin converting enzyme (ACE), ACE2, angiotensin II type 1 receptor (AT1R) and AT2R. The RAAS genes have been known to play a profound role in glucose metabolism and blood pressure regulation [38]. Gene polymorphism of RAAS may be responsible for the development of diabetes and its associated complications. The levels of angiotensin II (AngII), the main vascular peptide are elevated due to the activation of RAAS; resulting in glucose homeostasis and progression of DM through inhibition of signal transduction, reduction of glucose uptake, insulin resistance and pancreatic β-cell destruction by oxidative stress induction [39]. Angiotensinconverting enzyme (ACE) is responsible for the metabolism of a vasodilator bradykinin and conversion of angiotensin I to active angiotensin II. I/D polymorphism of Angiotensin-Converting Enzyme (ACE) have been studied for its association with diabetic nephropathy in diabetic patients [38].
Glucose Transporter (Glut) Family: GLUT proteins are the members of the major facilitator superfamily of membrane transporters. GLUT proteins are encoded by SLC2 genes and have been proposed as candidate genes for TII DM [40]. This is a family of structurally related proteins that facilitate the transport of monosaccharides, polyols and small carbon compounds across the cell membrane of eukaryotic cells [41]. About fourteen GLUT isoforms have been isolated and classified into three classes based on sequence similarity. Class 1 (GLUTs 1-4, 14); Class 2 (GLUTs 5, 7, 9 and 11); and Class 3 (GLUTs 6, 8, 10, 12 and HMIT) (41). GLUT 2, encoded by SLC2, is mainly expressed by the hepatocytes, intestinal absorptive cells, by proximal convoluted tubular cells of the kidney, pancreatic β-cells and neurons present in the brain and erythrocytes; while GLUT4, encoded by SLC4, is mainly restricted to tissues with insulin-stimulated glucose uptakes such as adipose tissue, skeletal muscle and cardiac muscles [40]. Variations in mRNA expression of these GLUTs are associated with the pathogenesis of TII DM.
Vitamin D: Studies have shown that vitamin D deficiency is associated with a decrease in insulin release, insulin resistance and TII DM [42]. It has also been shown that the risk of developing diabetes in adulthood decreases when vitamin D supplementations are taken during the early years of life [43]. Activation of vitamin D occurs when it is bound with its specific nuclear vitamin D Receptor (VDR) [44].When intracellular VDR is bound with the activated form of vitamin D; 1, 25(OH)зDз, it can enhance pancreatic β-cell function, protect β-cell from immune attack, enhance immune receptor sensitivity and reduce immune resistance [45]. Location of VDR gene is on chromosome 12q12-q14 and its genetic alterations could lead to defects in gene activation or changes in the VDR protein structure, affecting the cellular functions of vitamin D [45]. Glucose intolerance may develop due to high parathyroid hormone concentration which is enhanced when the deficiency of vitamin D causes secondary hyperparathyroidism. Insulin resistance could also develop through the increased inflammatory condition which is associated with vitamin D deficiency [42]. The anti-inflammatory effects of vitamin D improve islets cell function, aids in insulin release and decrease insulin resistance [46].
Endothelial Nitric Oxide Synthesis (eNOS): Diabetic nephropathy is the common causal factor for Chronic Kidney Disease (CKD), stroke and heart attack. It has been estimated that about 30% of the diabetic patients develop diabetic nephropathy [47]. Although many cell types are involved, evidence suggests that endothelial dysfunction serves as a major event in the development and progression of diabetic vascular complications, including nephropathy [48]. Multiple endothelial functions involve a critical role of Nitric Oxide (NO) produced by endothelial cells through eNOS. Endothelial dysfunction in diabetes could greatly arise as a result of a decrease in NO production and bioavailability [49]. Moreover, the results have also suggested that the combined effects of eNOS deficiency and hyperglycemia contribute to podocytes injury in diabetes [50] Table 1.
Table 1: Susceptibility loci associated with Type II DM. Showing the susceptible genes, gene region, single nucleotide polymorphisms (SNPs) of particular genes and their related function in correlation to Type II DM.
Table 1 Abbreviations: KCNJ11- potassium voltage gated channel subfamily J member 11, PPARG- Peroxisome proliferator activated receptor gamma, ABCC8- ATP Binding cassette subfamily C member 8, IRS1- insulin receptor substrate 1, TCF7L2- transcription factor 7 like 2, CAPN10- Calpain 10, KCNNQ11- potassium voltage gated channel KQT like subfamily member 1, IGF2BP2- Insulin like growth factor 2 MRNA binding protein 2, HHEX- hematopoietically expressed homeobox protein, JAZF1- juxtaposed with another zinc finger protein 1, ATR- Angitensin II type 1 receptor, AT2R- Angitensin II type 2 receptor, CDKN2A/B- Cyclin dependant kinase inhibitor-2A/B, MTNR1B- Melatonin receptor 1B, VDR- vitamin D receptor.
GDM
Based on the studies related to the candidate gene approach and genome-wide association study, it has been suggested that GDM share similar genetic background with TII DM since insulin resistance and defect in insulin secretion contribute in the etiology of both TII DM and GDM [17] such as CDK5 regulatory subunit associated protein 1 like-1 (CDKAL1), transcription factor 7-like2 (TCF7L2), hexokinase domain containing 1 (HKDC1), HNF4A has been associated with both TII DM and GDM (17, 52). Candidate gene approach has also shown that GDM also shares a similar genetic background with TI DM. HLA DR3 and DR4 antigens have been associated with GDM and have been found to be higher in women with GDM. Moreover, GCK variations significantly contribute to the risk of both GDM and MODY2 [52].
MODY
Genetic studies demonstrated that development of MODY may have many causes in addition to a single genetic entity. Mutations in HNF1A (MODY3) and GCK (MODY2) are the common causes of MODY and accounts for 30-60% of all MODY while mutations in HNF4A (MODY1), HNF1B (MODY5) and INS (MODY10) are its less commonly causes, accounting for about 10% of all MODY [53,54]. Other mutations including ABCC8 (MODY12), APPL1 (MODY14), BLK (MODY11), CEL (MODY8), KCNJ11 (MODY13), KLF11 (MODY7), NEURODY (MODY6), PAX4 (MODY9) and PDX1 (MODY4) accounts for less that 1% of all MODY [54].
FCPD
Recently reports have suggested increasing evidence of clustering of FCPD in families, indicating a possible genetic association of the disease [55]. DQ1, HLA-DQ9 and HLA-DQB1 allele are the potential candidate for FCPD. Studies have reported that in India, TAQ1 restriction fragment length polymorphism of DQ1 gene and have been associated with FCPD [22]. HLA-DQB 1 allele RFLP has been associated with FCPD pain in southern India and Bangladesh, similar to TI DM [22,56]. Major Histocompatibility Complex (MHC) has also been found to be associated with Bangladeshi subjects [56]. The hypervariable region of insulin gene (INS) has been associated with some FCPD patients possessing class 3 allele; similar to TII DM found in some ethnic group [57]. Thus, it can be said that FCPD shares the genetic susceptibility features similar and dissimilar to both TI DM and TII DM.
MIDD
Patients with MIDD show a strong family history of diabetes, deafness and retinal dystrophy, indicating that MIDD has a strong genetic association. Studies have shown that mutation in Mitochondrial DNA (mt-DNA) contribute to the development of MIDD since mitochondrial oxidative phosphorylation (OXPHOS) plays a crucial role in glucose-stimulated insulin secretion from pancreatic β-cell [58]. Mutations in mt DNA 3243A>G in tRNA is the cause of MIDD in upto 85% of the cases reported but other mt DNA mutations could also be the causal factor [27]. Premature aging of β-cells and reduction in insulin secretion is the prominent cause of diabetes development through this mutation [59] Table 2.
Table 2: Susceptibility loci of various diabetes types. Showing the genetic factors associated with Type I DM, Type II DM, Gestational DM, MODY, FCPD and MIDD.
Table 2 Abbreviations: HLA- Human leukocyte antigen, PTPN22- Protein tyrosine phosphate, non receptor 22, CTLA4- cytotoxic T lymphocyte associated protein 4, IL2RA- interleukin receptor 2 subunit alpha, WFS- Wolfram like syndrome, HNF- Hepato nuclear factor, AGT-Angiotensinogen, ACE- Angiotensin converting enzyme, SLC- Solute carriers, HKDC- Hexokinase domain containing 1, GCK- Glucokinase, INS- Insulin, APPL1- Adaptor protein phosphotyrosine interacting with PH domain and leucine zipper, BLK- B lymphocyte kinase, CEL- Carboxyl ester lipase, PAX- Paired box, MHC- Major histocompatibility complex.
A Need for Herbal Treatment?
Insulin therapy and synthetic anti-diabetic drugs such as thiazolidinediones and sulfonylureas are commonly used to reduce the glucose level in diabetic patients; however, their major limitation is that they often fail to maintain a normal glucose concentration range and desirable glucose homeostasis within the body [60]. These drugs often tend to develop resistance and loose effectiveness after a few years of their use. Continued use of insulin treatment could enhance the risk of hypoglycemia and arteriogenesis. A drug such as thiazolidinediones might develop liver toxicity; vigorous use of sulphonylureas may become the cause of hypoglycemia, weight gain and heart disorder; use of glucoside inhibitors over an extended period may be accompanied with bloating, flatulence, diarrhea and abdominal pain [61]. The increased incidence of diabetes, the inefficiency of synthetic medicines, their sky-reaching costs and their detrimental side effects have propagated an urgent need for the development of natural, indigenous, pocket- and health- friendly herbal products for the treatment and complete cure of diabetes.
Treatment of Diabetes with Herbal Plant Products
In addition to maintaining a normal glucose level and homeostasis, herbal products have also been proven for their ability to reduce and prevention of secondary complications which are accompanied with diabetes. Some plant based products are effective in overcoming resistance and in the regeneration of pancreatic β-cells. Although several medications and drugs are available to reduce the detrimental effects of diabetes and its complications, the limitations of these therapies are its high cost and serious side-effects [62]. Medicinal plants are being looked-up for their easy availability, lesser side-effects and cost-effective treatment. The hyperglycemic condition developed due to diabetes enhances the formation of free radicals which induce the modification of extracellular proteins such as elastin, laminin and collagen to form a glycoprotein. Diabetes-associated complications including cataract, microangiopathy, atherosclerosis and nephropathy develop when these extracellular proteins of lens, vascular walls and basement membrane are modified [63]. The pathophysiology of diabetes and related complications include the involvement of free radicalinduced damage and oxidative stress which suggests that medicinal plant with antidiabetic anti-inflammatory and antioxidant property could be the suitable treatment method to neutralize the effects of free radicals [63]. Many herbal drugs indigenous to different Asian countries were claimed as anti-diabetic by traditional medical practitioners. Despite the medicinal herbs, some of the plant based foods that are commonly consumed world-wide on a day-day basis such as tulsi, garlic, aloe vera, bitter melon, pumpkin, lotus root, wheat, tomato, celery, Jerusalem artichoke, soy bean, asparagus, beet root, blackberry, Indian mustard and yoghurt were also proved to possess anti-diabetic activity by scientific studies across the globe [63,72, 96,99,100,101,102].
In line with this an interesting randomized, double-blinded, placebo controlled clinical study shown the combinatorial effect of Jerusalem artichoke and fermented soybean powder mixture as anti-diabetic (64). An article published earlier reviewed the anti-diabetic nature of the Chinese herbs approved by the Chinese health regulatory agency for commercial use in China [65]. Among the approved plants those that are rich in polysaccharide (seven herbs) were able to restore the functions of pancreas and also increased the insulin output by functional beta cells (seven herbs). Further, herbs such as Pueraria lobata and Hirudo nipponia were reported to improve the microcirculation and thereby increase the availability of insulin and regulate the metabolism. Puerain an active component of Pueraria lobata was reported to treat cardiovascular complications and shown significant hypoglycemic activity in alloxan induced diabetic mice [65]. The major advantage of natural anti – diabetic agent over the synthetic drugs as antidiabetics is its lesser side effects. It also acts in synergy with conventional drugs and enhance its efficacy, thereby dosereduction, - frequency and -scheduling can be achieved without compromising the therapeutic outcome of the conventional drugs. Many Chinese herbs either as monotherapy or in combination were shown to reduce cholesterol and triglycerides levels; hence their long – term use may be advantageous to combat the diabetes and its associated complications than conventional drug(s). In addition to these Chinese herbs some of the Indian spices and herbs were also reported to possess anti-diabetic activity. For example, 4-hydroxyleucine, an amino acid present in seeds of Trigonella foenum graecum (fenugreek), one of the major spices of India, was reported to enhance the glucose-stimulated insulin release by islet cells in both pre-clinical and clinical trials. A dose-dependent reduction in the blood glucose level in both normal and diabetic rats has been found on oral administration of 2-8 g/kg of fenugreek plant extract [63,66].
Cinamomi cassiae (cinnamon bark) is one of the traditional herbs for the treatment of diabetes and other ailments in many countries over the world due to its anti-diabetic, anti-inflammatory, antibacterial and antioxidant properties. The major components of cinnamon include cinnamic aldehyde, cinnamic acid, tannin and methylhydroxychalcone polymer [67]. Recent researches on cinnamon have found that cinnamon extract decreases blood glucose in Wistar rats. Evidence also indicates that cinnamon increases insulin sensitivity and glucose uptake in adipocytes [67]. Studies have suggested that A-type doubly linked procyanidine oligomers of the catechins and/or epicatechins from cinnamon may show insulin-enhancing properties in vitro in adipocytes [68]. One of the probable mechanisms for the hypoglycemic effect of cinnamon is that cinnamon increases glucose entry into cells by enhanced tyrosine phosphorylation of (IR)-β, insulin receptor substrate with Phosphotidylinositol (PI) 3-kinase and GLUT4 translocation to the plasma membrane [69,68]. Syzygium cumuni commonly known as jamun belongs to the family Myrtaceae. Ayurvedic pharmacopedia has acknowledged the anti-diabetic effect of jamun seeds. According to it the seed powder has a significant effect in controlling blood sugar level [70]. Various studies have indicated that several bioactive antioxidant compounds including phenolic acids, flavonoids and anthocyanins are present in a notable amount in the fruits and seeds of jamun which have a role in preventing metabolic syndromes [71]. These bioactive compounds also help in regulating lipid blood profile due to which jamun show antihypercholesterolemic properties [71].
Studies have reported that supplementation of jamun seed powder in human diabetic subjects helps in reducing both fasting and postprandial blood glucose levels. Another study conducted on aqueous extract of jamun seeds showed a significant reduction in blood sugar level, an increase in total hemoglobin and decreased free radical formation [70]. Allium sativum (garlic), a perennial herb cultivated in India and is also one of the major components of Indian spices. Studies have indicated that a sulphur containing amino acid, S-allyl cystine sulphoxide (SACS) which is the precursor of allicin and garlic oil show antidiabetic effects in alloxan diabetic rats. SACS control lipid peroxidation, serum lipids, blood glucose several enzymatic activities and invitro insulin secretion from beta cells isolated from normal rats [65,72]. The hypoglycemic activity of allicin can be explained due to increased hepatic metabolism, increased insulin release from beta cells and/or insulin-sparing effect. The anti-inflammatory, anti-oxidative and anti-glycative effects of garlic may also benefit from the complications and disorders associated with diabetes such as cardiovascular diseases, diabetes nephropathy, activated coagulation, hypertension, dislipidemia and obesity [73]. In addition to herbs normal food including yoghurt were shown to be anti-diabetic. For instance, a study compared the prophylactic activity of conventional yoghurt vs. probiotic yoghurt enriched with bacteria (Lactobacillus acidophilus LA5 and Bifidobacterium animalis-BB12) in a randomized controlled clinical trial involving 70 primigravida-singleton pregnant women revealed that consumption of probiotic yoghurt for 9 weeks daily was much better in preventing the development of insulin resistance in pregnant women than conventional yoghurt [74]. Similarly, another study demonstrated the daily intake of yogurt to reduce the risk of diabetes by 14% on comparison with the group that did not consumed yogurt. Further a review article published earlier analyzed 13 different prospective studies that evaluated the role of yogurt in lowering the risk of diabetes (Table 3). Cumulative analysis of several reviews and research articles clearly states the significance of daily intake of yogurt could be a healthy dietary habit to reduce the TII DM and its associated complications (Figure 3).
Table 3: Herbal plants with anti-diabetic and other beneficial properties. Showing botanical names of plants along with their common names and their anti-diabetic and other beneficial uses.
Figure 3: A summary of influencing factors of diabetes and role of herbal products in prevention of diabetesindicate inhibition
indicate stimulation.
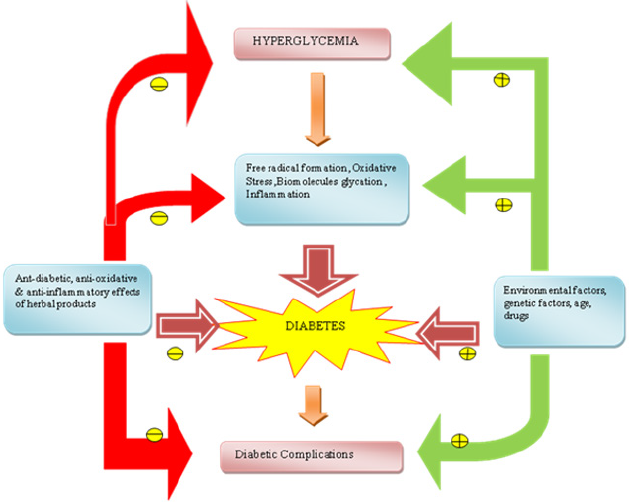
Conclusion
DM is not only a disease in itself but is also the root cause of other deadly diseases including cancers, heart and kidney related diseases. The disease is ruining the health of almost all ethnicities with the low and middle income groups being its prime targets. Knowing the different context in which DM and its variants could occur and understanding about the underlying causes and their genetic correlation would definitely help in an early diagnosis and subsequent planning of rapid and adequate treatment strategy. Moreover, an early diagnosis would also combat the serious diabetes related complications which are accompanied with diabetes. The economic condition of a common man and the strenuous side effects of some medications have has urged the need for the adoption of effective herbal treatment strategy. The herbal treatment could not only help to combat diabetes and related complication but may also prove to be a complete cure of this pandemic disease.
To Know More About Open Access Publishers Please Click on Lupine Publishers
Follow on Linkedin : https://www.linkedin.com/company/lupinepublishers
Follow on Twitter : https://twitter.com/lupine_online
Wednesday, September 9, 2020
Lupine Publishers: Lupine Publishers | Effects of Urban Farming Pract...
Tuesday, September 8, 2020
Lupine Publishers: Lupine Publishers | Effects of Urban Farming Pract...
Lupine Publishers| Potentiation of Activity of Benfotiamine Co Administered with Thyroxine in Diabetes Induced Peripheral Neuropathy in Rats
Lupine Publishers| Potentiation of Activity of Benfotiamine Co Administered with Thyroxine in Diabetes Induced Peripheral Neuropathy in Rats
Abstract
Diabetic peripheral neuropathy (DPN) is a multi-etiological microvascular complication. Prolong hyperglycemia leads to formation of advance glycation end product (AGE) and oxidative stress which are contributors of nerve dysfunction. DPN manifests as pain, slowing of nerve conduction velocity (NCV), sensory loss etc. The aim of the present study is to evaluate the individual and combined protective effect of benfotiamine (BT) and thyroxine (T4) against Streptozotocin (STZ) induced DPN in rats. After 48 hours of a single injection of STZ (60 mg/kg bw i.p) diabetic rats were administered BT (100 mg/kg p.o.), T4 (1mg/kg.s.c,) and their combination. Diabetic rats at 5th week, exhibited significant decrease in body weight, hyperalgesia, decreased muscle coordination, grip strength and NCV. Antioxidant activity of reduced glutathione (GSH), catalase (CAT), superoxide dismutase (SOD) was also found to be significantly decreased. Significant higher levels of glycosylated hemoglobin (GHb) and Malondialdehyde (MDA) were also observed in diabetic rats. Treatment with BT, T4 and their combination attenuated the decrease in level of nociceptive threshold, muscle coordination, grip strength.
NCV and antioxidant activity. Significant decrease in the elevated levels of GHb and MDA was also observed. A histopathological study of sciatic nerve also confirmed the improvement in cell architecture as compared to diabetic rats and has strengthened the neuroprotective effect of BT and T4 combination group. An improved In Vitro AGE inhibitory activity of BT, T4 and their combination was observed. These finding suggested that BT, T4 and their combination exerts a protective effect in progression of diabetic neuropathy by decreasing GHb, AGE formation and oxidative stress.
Keywords:Micro vascular; NCV; Antioxidant; AGE; Thyroxine; Benfotiamine
Abbreviations:DM: Diabetes mellitus; DPN: Diabetic Peripheral Neuropathy; MAPK: Mitogen Activated Protein Kinase; NCV: Nerve Conduction Velocity; TH: Thyroid Hormones; BT: Benfotiamine; AGE: Advance Glycation End Product; LPO: Lipid Peroxidation; SOD: Superoxide Dismutase; CAT: Catalase; GSH: Glutathione; TBARS: Thiobarbituric Acid Reactive Substances; BSA: Bovine Serum Albumin; PKC: Protein Kinase C; ROS: Reactive Oxygen Species
Introduction
Diabetes mellitus (DM) is a worldwide major health problem and it is a chronic metabolic disorder characterized by hyperglycemia resulting from inadequate secretion or impaired action of endogenous insulin. The prevalence of diabetes is increasing worldwide and is believed to increase to 300 million by the year 2030 [1]. Uncontrolled or persistent hyperglycemia in DM leading to several microvascular and microvascular complications. Diabetic peripheral neuropathy (DPN) is a common microvascular complication of DM affecting more than 50% of the diabetic patients [2]. The pathogenesis of DPN is considered to be complex and multifactorial resulting from contributions of various pathways including metabolic and vascular factors, which consists of activation of polyol pathway, advanced glycation end products pathway, hexosamine pathway, increased activity of mitogen-activated protein kinase (MAPK), protein kinase C, poly (ADP-ribose) polymerase, oxidative stress, apoptosis, impaired neurotrophic support, autoimmunity, inflammation, up regulation of endothelin [3]. The impairment of nerve function is a well-established early manifestation of diabetes both clinically and in experimental animal models. DPN causes dysfunction of small and large nerve fibers and negatively impacts quality of life in diabetic patients. Small-fiber peripheral neuropathy is characterized by behavioral abnormalities (cold, thermal hyperalgesia, loss of grip strength and motor incoordination and burning or lancinating pain, and predisposition to foot ulceration). Large-fiber dysfunctions include loss of position and vibration sensation, nerve-conduction abnormalities, and distal muscle weakness. Early disorders of nerve function include slowing in nerve conduction velocity (NCV), followed by axonal degeneration, axoglial disjunction, paranodal and loss of fibre density. Microangiopathy [4]. A number of different agents from diverse chemical classes have entered clinical trials for the treatment of metabolic abnormalities in DPN, but only few approved for clinical use while other drugs either ineffective or withdrawn [2]. Current treatment options for symptomatic treatment of DPN include antidepressants, anticonvulsants. These agents are modestly effective for symptomatic relief, but they neither affect the underlying pathology nor do they slow progression of the disease [5]. Hence a novel approach to bridge the gap in selecting the compound in treatment of DPN was used .The discovery of use of a drug for a new indication is an arbitrary process, as shown by many past examples like the use of zinc acetate for the treatment of Wilson’s disease [6], arsenic for acute promyelocytic leukemia [7], amphotericin B for leishmaniasis [8], and thalidomide for multiple myeloma [9]. The discovery of these “alternative” uses for drugs different from originally intended drug development process is referred to as drug repurposing or repositioning. Repositioning of drug efforts has many advantages, because the pharmacokinetics and pharmacodynamics of the drug are known, repositioning discoveries are less costly and quicker than traditional discovery efforts [10], which usually take 10-15 years [11], and cost upward of $1 billion [12], Although physicians and pharmaceutical/ biotechnology companies have manual methods and prior knowledge that enable them to carry out drug repositioning clinical trials, such successful repositioning of drugs is often serendipitous and rare. In this study we have selected thyroxine to explore for its activity in DPN. Thyroid hormones (TH) [T4 (tetraiodothyronine) and T3 (triiodothyronine)], the only iodine-containing compounds with biological activity [13]. TH stimulate synthesis of Na+/K+ ATPase and also regulates metabolism by stimulating protein synthesis and increase the use of glucose and fatty acids for ATP production. They also increase lipolysis and enhance cholesterol excretion [14]. The cardiac side effect of D isomer of thyroxine resulted discontinuation of the clinical uses of this hormone. Under normal conditions, about 41%of thyroxine is converted to T3, and about 21% is converted to metabolically inactive 3,3,5-triiodothyronine (reverseT3, rT3). T3 is a powerful inducer of pancreatic acinar cell proliferation in rodents [15]. In Vitro studies of human and rodent insulinoma cell lines showed that T3 protected from apoptosis and induces β-cell growth and proliferation [16]. A serendipitous, positive association between serum-FT3 (free tri iodothyronine) and an estimate of insulin production was found in euthyroid, lean, healthy individuals [17].Treatment of Human pancreatic duct cells (hPANC-1)with T3 induced changes in cell morphology, promotes cell differentiation into insulin-producing β-cells, unregulated insulin and glucose transporter protein-2 transcripts, and increases insulin release into the medium. TH receptor has been identified in pancreatic β -cell lines [18,19], T3-enhanced insulin release in isolated rat pancreatic islets exposed to glucose concentrations of 2-8 mmol/l, had no effect at concentrations of 12 mmol/l, and inhibited insulin release at concentrations of 16.6 mmol/l [20]. T3 promoted expression of important proteins involved in both glucose and lipid metabolism that may influence insulin secretion [21]. Benfotiamine (BT), a lipid soluble vitaminB1 with much higher bioavailability than thiamine [5] Benfotiamine was shown to prevent experimental diabetic retinopathy and In Vitro hyperglycemia-induced endothelial dysfunction. The effects of benfotiamine on in vivo endothelial function remained unknown [22]. Therefore, the present study was designed to evaluate whether diabetes induced DPN can be reversed by treatment with thyroxine and BT.
Materials and Methods
Experimental Animals
In-house laboratory bred healthy Wistar rats of weighing 200-250g were included for the study. Animals were housed in polypropylene cages on clean paddy husk bedding. Animals were maintained under controlled temperature at 25°C± 2°C with 12hr light/dark cycle with food and water provided ad labium. Animals which do not comply with above criteria, and which are found to be diseased will be excluded from the study. Before conducting the experiment, ethical clearance was obtained from “Institutional animal ethics committee” Al-Ameen College of Pharmacy”, Bangalore. Approval No: AACP/P-48.
Drugs and Reagents
Thyroxine (gift sample from Apotex Pharmachem India Pvt. Ltd.), benfotiamine (gift sample from Strides Arco Lab Pvt.Ltd.) were used in the present investigation. Streptozotocin (STZ) was purchased from Sigma Aldrich. Commercial diagnostic kit for the estimation of serum glucose was obtained from Span Diagnostics Ltd. Glycosylated hemoglobin kit was obtained from Crest Biosystems Pvt. Ltd. Other chemicals and reagents were of analytical grade and purchased from local suppliers.
Design of the Experiment
Induction of Diabetic Peripheral Neuropathy: After an overnight fast, Wistar rats were administered a single injection of streptozotocin [60 mg/kg of body weight i.p.in 100 mM sodium citrate buffers, pH 4.5] [23]. After 48 hours, animals with fasting blood glucose levels higher than 250mg/dl were selected for the study.
Experimental Procedure
Rats were randomly assigned in six groups (n=6)
Group1: Normal Control
Group2: Diabetic Control
Group3: Diabetic Control+ T4 (1mg/kg.s.c) [24] thrice a week for 5 weeks
Group4: Diabetic Control+ BT (100 mg/kg p.o.) [25] daily for 5 weeks
Group5: Diabetic Control +BT (100 mg/kg p.o.)+T4 (1mg/ kg.s.c,) for 5 weeks
For preventive studies treatment was started from day 2 of STZ administration along with insulin (3IU/kg, s.c) [26]. 5 weeks post treatment various behavioral, biochemical, electrophysiological and histopathological parameters were studied.
Behavioral Studies
Thermal and Cold Hyperalgesia [27,28]: Thermal and cold hyperalgesia were measured using the tail-immersion test in water maintained at high (46ºC) or low (4ºC) temperature. The duration of tail immersion was recorded, and a cut-off time of 15 s was used.
Measurement of Muscle Incoordination Using Rota Rod [28,29]: Rota rod has been used to evaluate motor coordination by testing the ability of rats to remain on a revolving rod. The apparatus has a horizontal rough metal rod of 3 cm diameter attached to a motor with variable speed. This 70 cm long rod was divided into four sections by wooden partitions. The rod was placed at a height of 50 cm to discourage the animals to jump from the rotating rod. The rate of rotation was adjusted in such a manner that it allowed the normal rats to stay on it for 5 min. Each rat was given five trials before the actual reading was taken. The readings were taken at15, 25, rpm after treatment in all groups of rats.
Measurement of grip strength [29,30]: Grip strength meter was used for evaluating grip strength of animals. Before commencement of experiment, the animals were acclimatized by placing on the instrument for some time to train, and then rats were held by the tail above the grid of grip strength meter. The animal was moved until its front legs were grasped the grid and it was brought to an almost horizontal position. The base of the tail was then pulled following the axle of the sensor until it released the grid. The force achieved by the animal was then displayed on the screen and was recorded as new tons or kg units.
Biochemical Studies
Estimation of GHb [30,31]: At the end of study (5 weeks) blood was withdrawn through retroorbital plexus of overnight fasted rat under light ether anesthesia using a glass capillary and collected in EDTA tubes. The glycosylated hemoglobin was determined by using kit. A hemolysed preparation of whole blood is mixed continuously for 5 minutes with a weakly binding cation-exchange resin. The labile fraction is eliminated during the hemolysate preparation and during the binding. During this mixing, HbAo binds to the ion exchange resin leaving GHb free in the supernatant. After the mixing period, a filter separator is used to remove the resin from the supernatant. The percent GHb is determined by measuring absorbance of the GHb fraction & the total hemoglobin (THb) fraction. The ratio of the absorbance of the GHb & the THb fraction of the Control and the Test is used to calculate the percent GHb of the sample using below formula.
Preparation of Nerve Homogenate: A segment of sciatic nerve, approximately 1.5 cm in length, 5mm proximal and 5 mm distal was used for preparing the 10% w/v homogenates for biochemical estimation. Tissue homogenates were prepared in 0.1M phosphate buffer (pH 7.4). The homogenate was centrifuged at 1000 rpm 4ºC for 3 min and the supernatant divided into two portions, one of which was used for measurement of lipid peroxidation (LPO) and the remaining supernatant was again centrifuged at 12,000 rpm at 4ºC for 15 min and used for the measurement of lipid peroxidation (LPO), superoxide dismutase (SOD), catalase (CAT) and glutathione (GSH).
Measurement of Lipid Peroxidation: The extent of lipid peroxidation in terms of thiobarbituric acid reactive substances (TBARS) formation was measured according to the method of Esterbauer and Cheeseman. Tissue extracts were mixed separately with 1ml TCA (20%), 2ml TBA (0.67%) and heated for 1h at 100°C, after cooling, the precipitate was removed by centrifugation. The absorbance of each sample was measured at 535 nm using a blank containing all the reagents except the sample. As 99% TBARS are malondialdehyde (MDA), so TBARS concentrations of the samples were calculated using the extinction coefficient of MDA, which is1.56 x 105 M-1 cm-1 and were expressed as μM of malondialdehyde per mg protein [31-34].
Estimation of Superoxide Dismutase (SOD) and Catalase Activity: Sciatic nerve homogenate was centrifuged at 4°C, 17,500×g for 10min. Supernatant was used for the measurement of SOD activity by pyrogallol autooxidation method [35,32] and catalase activity by H2O2 degradation method, which is a quantitative spectroscopic method developed for following the breakdown of H2O2 at 240nm in unit time. The sample readings were taken by placing 1ml of phosphate buffer and 100 μl of tissue homogenate in the reference cuvette and 1 ml of H2O2 and 100 μl of homogenate in the test cuvette in the spectrophotometer. For each measurement, the reading was taken at 240nm 1min after placing the cuvettes in Shimadzu spectrophotometer [36,33].
Measurement of Reduced Glutathione Activity: Reduced glutathione was assayed by the method of Van Dooran [37,34]. Briefly1.0 ml of sciatic nerve homogenate (10%w/v) was precipitated with 1.0 ml of sulphosalicylic acid (4%). The samples were kept at 4°C for at least 1h and then subjected to centrifugation at 1200g for 15min at 4°C.The assay mixture contained 0.1 ml supernatant,2.7 ml phosphate buffer (0.1M, pH 7.4) and 0.2 ml 5,5, dithiopyrs (2-nitro benzoic acid) (Ellman’s reagent, 0.1 mM, pH 8.0) in a total volume of 3.0 ml. The yellow color developed was read immediately at 412nm and the reduced GSH levels were expressed as μg/mg protein.
Electrophysiological Studies
Isolation of Sciatic Nerve: The rats were sacrificed by administration of overdose of ketamine/xylazine i.p. After anesthesia, rat backs were shaved and NCV was recorded. Briefly incision was made at L4-L6 spinal segments. The sciatic nerves were surgically exposed from sciatic notch to the gastrocnemius tendon and the left and right sciatic nerves were rapidly removed, carefully impregnated on fine filter paper to remove any accompanying blood soaked for 10 minutes in Ringer-Locke buffer to prevent spontaneous firing of the nerve.
Measurement of Nerve Conduction Velocity (NCV): The rats were anesthetized by administration of thiopentone sodium, 30mg/kg, and i.p [1]. After anesthesia, rat backs were shaved and motor NCV was recorded. Briefly incision was made at L4-L6 spinal segments. The sciatic nerves were surgically exposed from sciatic notch to the gastrocnemius tendon and the left and right sciatic nerves were rapidly removed, carefully impregnated on fine filter paper to remove any accompanying blood soaked for 10 minutes in Ringer-Locke buffer to prevent spontaneous firing of the nerve [32].The left sciatic nerves were then placed in a moist nerve chamber (MLT016/B AD Instruments, Australia) to measure NCV. NCV was measured by stimulating proximally at the sciatic notch by stimulating electrode (MLA270 AD Instruments, Australia) with 10 mV at 1Hz to 5 Hz and the action potential was measured using recording electrodes (MLA 285) by placing distally to the sciatic knottins was calculated by distance between stimulating and recording electrodes divided by the latency. Right sciatic nerves were transferred into Glutaraldehyde solution for histopathological studies and rinsed with ice cold saline homogenized in chilled phosphate buffer (pH 7.4) and used to assay lipid peroxidation, reduced glutathione and catalase [33,35].
Histopathological Studies
The right sciatic nerves were isolated and transferred in to 0.05mol/L phosphate buffered (30g/L) glutaraldehyde solution for histopathological studies (H&E staining, Kulchitsky Pal staining and Massion’s trichome) [36,37].
To determine In Vitro glycation of protein bovine serum albumin (BSA)-glucose assay was performed based on the procedure of Brownlee et al. BSA (l0mg/mL) was incubated with glucose (500mM) in phosphate buffered-saline (pH 7.4) and extract containing sodium azide (0.02%) at 37ºC with a final concentrations of BSA (2mg/mL), glucose (40mM), sample (0.1 to 0.5mg/mL). Sterilization of reagents and samples were done by filtration through 0.2μm membrane filters. Aminoguanidine was used as an inhibitor positive control and a reactions without any inhibitor were also setup. All the samples and positive control were kept for incubation at 37ºC for 15 days. After 15 days of incubation, fluorescence intensity (excitation wavelength of 370nm and emission wave-length of 440nm) was measured for the test solutions. Percent inhibition was calculated as follows: Inhibition %= Inhibition % = (1− ( As − Ab) ( Ac − Ab) ×100
Where As = fluorescence of the incubated mixture with sample, Ac, Ab = are the fluorescence of the incubated mixture without sample as a positive control and the fluorescence of incubated mixture without sample as a blank control.
Statistical Analysis
Statistical evaluations were done by ANOVA, expressed as mean± S.E.M. followed by Bonferroni comparison test using Graph Pad In Stat (Ver.3.10) and Graph Pad Prism 5 computer programme.
Results
Assessment of General Toxicity
The percentage body weight of normal and diabetic rats treated with T4, BT and combination at 5th week was found to be 26.87±1.74g, -15.90 ± 0.769g,-6.433±0.493g.-6.635 ± 0.661,-5.150± 0.4366.The percentage of change of body weight of diabetic rats significantly less (<P0.001) as compared to normal control, similarly the percentage of change of body weight of diabetic treated rats was significantly less (<P0.001) as compared to diabetic control rats control (Figure 1).
Figure 1: Effect of treatment of thyroxine, benfotiamine and their combination for five weeks on %body weight change in diabetic rats. NC: normal control, DC: diabetic control, T4: thyroxine, BT: benfotiamine COM: combination of thyroxine and benfotiamine. Values are represented as mean ± SEM (n=6). ### P<0.001 Vs normal control group, and *** P<0.001 Vs diabetic control group. One Way ANOVA followed byBonferroni multiple comparisons.
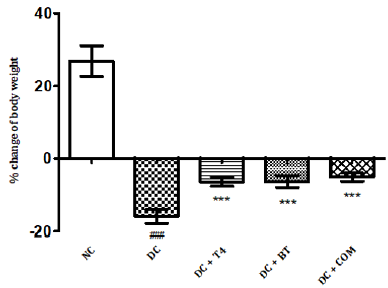
Behavioral Studies
Thermal and Cold Hyperalgesia
The tail flick latencies in both hot and cold immersion test of diabetic rats significantly changed at 5th week in diabetic rats as compared to normal rats (P<0.001) . 5 weeks treatment with T4, BT and combination significantly improved P<0.001 cold and hot immersion performance. (Figures 2&3).
Figure 2: Effect of treatment of thyroxine, benfotiamine and their combination for five weeks on tail flick latencies (46oC) in diabetic rats. NC: normal control, DC: diabetic control, T4: thyroxine, BT: benfotiamine COM: combination of thyroxine and benfotiamine. Values are represented as mean ± SEM (n=6). ### P<0.001 Vs normal control group, *** P<0.001 Vs diabetic control group. One Way ANOVA followed byBonferroni multiple comparisons.
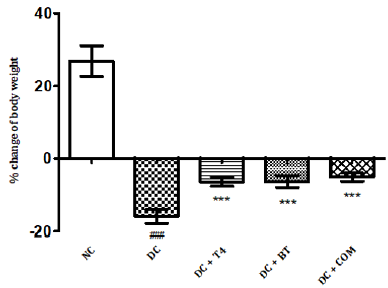
Figure 3: Effect of treatment of thyroxine, benfotiamine and their combination for five weeks on tail flick latencies (4oC) in diabetic rats. NC: normal control, DC: diabetic control, T4: thyroxine, BT: benfotiamine COM: combination of thyroxine and benfotiamine. Values are represented as mean ± SEM (n=6). ### P<0.001 Vs normal control group, *** P<0.001 Vs diabetic control group. One Way ANOVA followed by Bonferroni multiple comparisons.
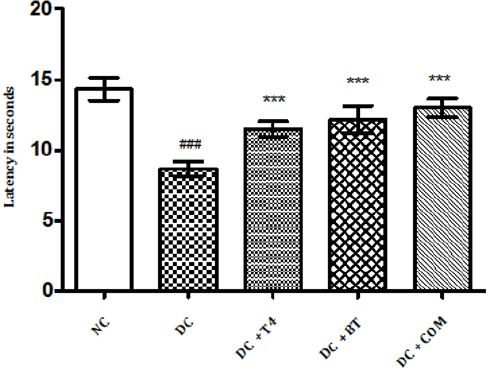
Measurement of Muscle Incoordination using Rota Rod
Time latencies at both 15, 25 rpm of normal rats was found to be 103.8±1.74,67.67±1.687 respectively, time latencies of diabetic rats at both 15,25 rpm was found to 75.5±1.176 38.83±0.307 and same were significantly reduced (P<0.001) as compared to normal control. Time latencies of diabetic rats treated with T4, BT and Combination at both 15, 25rpm was found to be 86.67±0.7149, 44.83±0.477, 86.83±0.792, 52.17±0.872, 91.5±0.846, 57.17±0.792 respectively and same were significantly P<0.001 improved (Figures 4&5).
Figure 4: Effect of treatment of thyroxine, benfotiamine and their combination for five weeks on muscle incordination by rota rod performance (15rpm) in diabetic rats. NC: normal control, DC: diabetic control, T4: thyroxine, BT: benfotiamine COM: combination of thyroxine and benfotiamine. Values are epresented as mean ± SEM (n=6). ### P<0.001 Vs normal control group *** P<0.001 Vs diabetic control group. One Way ANOVA followed by Bonferroni multiple comparisons.

Figure 5: Effect of treatment of thyroxine, benfotiamine and their combination for five weeks on muscle incoordination by rota rod performance (25rpm) in diabetic rats. NC: normal control, DC: diabetic control, T4: thyroxine, BT: benfotiamine COM: combination of thyroxine and benfotiamine. Values are represented as mean ± SEM (n=6). ### P<0.001 Vs normal control group, **P<0.01, and *** P<0.001 Vs diabetic control group. One Way ANOVA followed byBonferroni multiple comparisons.
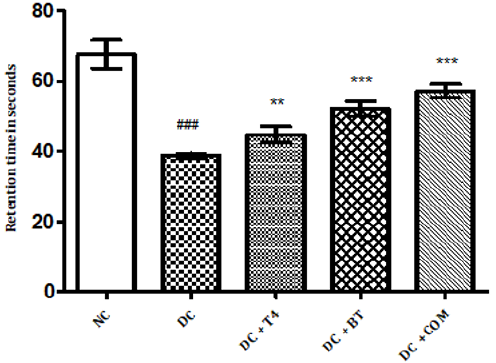
Measurement of Grip Strength
The grip strength of normal rats was found to be 9.822±0.1332,27.97±0.1171 respectively, The grip strength of diabetic rats was found to be 75.5±1.176, 38.83±0.307 and same were significantly reduced (P<0.001) as compared to normal control. Time latencies of diabetic rats treated with T4, BT and combination at both 15,25 rpm was found to be 86.67±0.7149, 44.83±0.477,86.83±0.792,52.17±0.872,91.5±0.846,57.17±0.792 respectively and same were significantly P<0.001 improved (Figure 6).
Figure 6: Effect of treatment of thyroxine, benfotiamine and their combination for five weeks on grip strength in diabetic rats. NC: normal control, DC: diabetic control, T4: thyroxine, BT: benfotiamine COM: combination of thyroxine and benfotiamine. Values are represented as mean ± SEM (n=6). ### P<0.001 Vs normal control group, *** P<0.001 Vs diabetic control group. One Way ANOVA followed byBonferroni multiple comparisons.
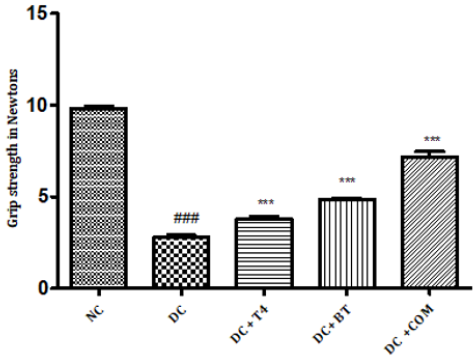
Biochemical Studies
Estimation of GHb: The percentage of GHb of normal rats was found to be 4.577±0.0249, The percentage of GHb of diabetic rats was found to be 9.537±0.066, and same was significantly reduced ( P<0.001) as compared to normal control. The percentage of GHb of diabetic rats treated with T4, BT and combination was found to be 9.357±0.01838, 5.698±0.02561, 5.277±0.0261, respectively and same were significantly improved. P<0.05 when compared T4 treated diabetic rats with diabetic control. P<0.001 when compared BT, combination treated diabetic rats with diabetic control (Figure 7).
Figure 7: Effect of treatment of thyroxine, benfotiamine and their combination for five weeks on % GHb in diabetic rats. NC: normal control, DC: diabetic control, T4: thyroxine, BT: benfotiamine COM: combination of thyroxine and benfotiamine. Values are represented as mean ± SEM (n=6). ### P<0.001 Vs normal control group,* P<0.05, and *** P<0.001 Vs diabetic control group.
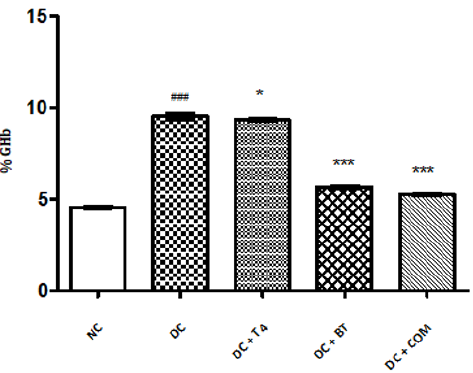
Measurement of Lipid Peroxidation: The sciatic nerve MDA levels of normal rats was found to 1.627±0.008433, The sciatic nerve of MDA levels diabetic rats was found to be significantly high (P<0.001) i.e 3.553±0.02860, The sciatic nerve MDA levels of diabetic rats treated with T4, BT and combination was found to be 3.235±0.008466, 3.368±0.009098, 4.080±0.01807, respectively and same were significantly (P<0.001) reduced (Figure 8).
Figure 8: Effect of treatment of thyroxine, benfotiamine and their combination for five weeks on sciatic nerve LPO in diabetic rats. NC: normal control, DC: diabetic control, COM: combination of thyroxine and benfotiamineValues are represented as mean ± SEM (n=6). ### P<0.001 Vs normal control group, ***P<0.001, Vs diabetic control group. One Way ANOVA followed byBonferroni multiple comparisons.
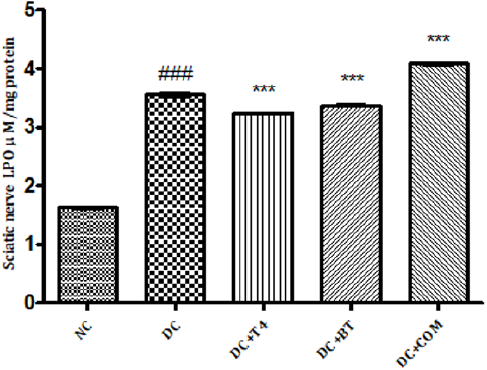
Estimation of Superoxide Dismutase (SOD): Sciatic nerve SOD activity in normal rats was found to be 205.7±0.1078, Sciatic nerve SOD activity was significantly (P.001) low 106.8±0.2798 in 5 weeks diabetic rats. SOD activity of diabetic rats treated with T4, BT and combination were found to be 130.0±0.2540, 135.6±0.1474 and 128.5±3.212 treatment significantly (P<0.001) restored SOD activity when compared to diabetic control (Figure 9).
Figure 9: Effect of treatment of thyroxine, benfotiamine and their combination for five weeks on sciatic nerve SOD in diabetic rats. NC: normal control, DC: diabetic control, T4: thyroxine, BT: benfotiamine COM: combination of thyroxine and benfotiamine. Values are represented as mean ± SEM (n=6). ### P<0.001 Vs normal control group, ***P<0.001, Vs diabetic control group. One Way ANOVA followed byBonferroni multiple comparisons.
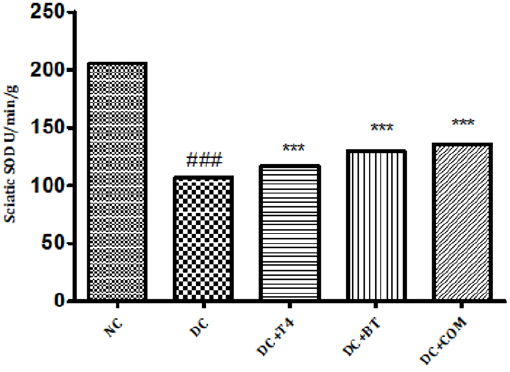
Estimation of Catalase: Sciatic nerve catalase activity in normal rats was found to be 0.1058± 0.0004, Sciatic nerve catalase activity was significantly (P.001) low 0.0545±0.0013 in 5 weeks diabetic rats. Catalase activity of diabetic rats treated with T4, BT and combination were found to be 0.08833±0.00230, 0.0950±0.00096and 128.5±0.00047 treatment significantly (P<0.001) restored catalase activity when compared to diabetic control (Figure 10).
Figure 10: Effect of treatment of thyroxine, benfotiamine and their combination for five weeks on sciatic nerve catalase in diabetic rats. NC: normal control, DC: diabetic control, T4: thyroxine, BT: benfotiamine COM: combination of thyroxine and benfotiamine. Values are represented as mean ± SEM (n=6). ### P<0.001 Vs normal control group, ***P<0.001, Vs diabetic control group. One Way ANOVA followed byBonferroni multiple comparisons.
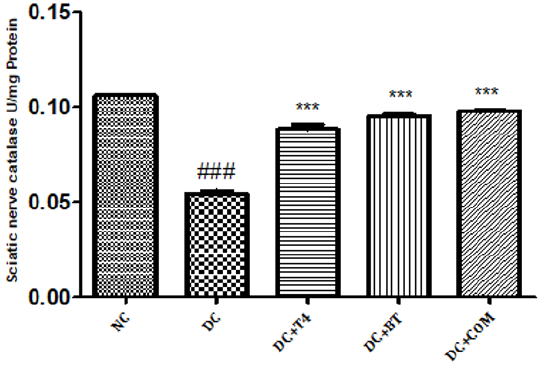
Measurement of Reduced Glutathione Activity: Sciatic nerve glutathione content in normal rats was found to be 0.1058± 0.0004, Sciatic nerve catalase activity was significantly (P.001) low 0.0545±0.0013 in 5 weeks diabetic rats. Catalase activity of diabetic rats treated with T4, BT and combination were found to be 0.08833±0.00230, 0.0950±0.00096and 128.5±0.00047 treatment significantly (P<0.001) restored catalase activity when compared to diabetic control. (Figure 11).
Figure 11: Effect of treatment of thyroxine, benfotiamine and their combination for five weeks on sciatic nerve GSH in diabetic rats. NC: normal control, DC: diabetic control, COM: combination of thyroxine, and Benfotiamine Values are represented as mean ± SEM (n=6). ### P<0.001 Vs normal control group, ***P<0.001, Vs diabetic control group. One Way ANOVA followed by Bonferroni multiple comparisons.
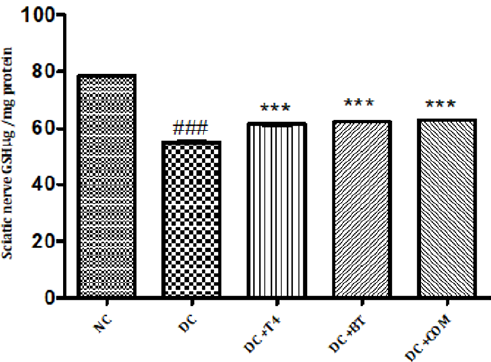
Measurement of Nerve Conduction Velocity (NCV)
Sciatic nerve conduction velocity was significantly (<P0.001) was significantly reduced in 5 weeks diabetic rats 44.11± 0.2907 when compared to normal 53.13±0.4599, T4 treated diabetic rats significantly (P<0.01) exhibited improved NCV 45.72±0.1954. BT and combination both have also significantly improved (P<0.001) NCV 48.01±0.1954, 48.64±0.1876 (Figure 12).
Figure 12: Effect of treatment of thyroxine, benfotiamine and their combination for five weeks on nerve conduction velocity in diabetic rats. NC: normal control, DC: diabetic control, T4: thyroxine, BT: benfotiamine COM: combination of thyroxine and benfotiamine. Values are represented as mean ± SEM (n=6). ### P<0.001 Vs normal control group, **P<0.01, and P<0.001 Vs diabetic control group. One Way ANOVA followed byBonferroni multiple comparisons.
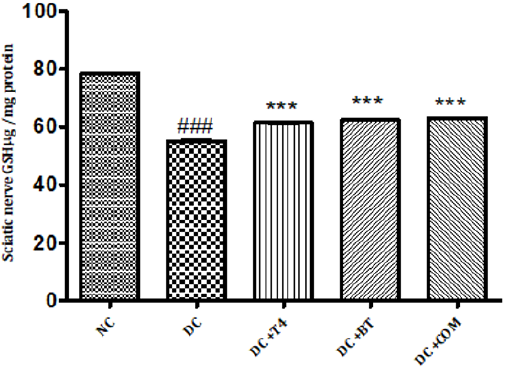
In Vitro Glycation of Proteins
AGEs formation after incubation at 37ºC for 15 days, with an IC50 value of BT, T4, combination and standard aminoguanidine was found out to be 166.6±0.45μg/ml,410.25±0.32μg/ml, 162.7±0.37 μg/ml, 322.4± 2.23 μg/ml respectively. The combination exhibited higher inhibitory activity i.e.162.7±0.37μg/ml against AGEs formation after incubation compare to aminoguanidine (Figure 13).
Figure 13: Effect of thyroxine, benfotiamine and their combination on in-vitro AGE inhibition. NC: normal control, DC: diabetic control, COM: combination of thyroxine and benfotiamine. Values are represented as mean ± SEM (n=6). ### P<0.001 Vs normal control group, **P<0.01, and *** P<0.001 Vs diabetic control group. One Way ANOVA followed byBonferroni multiple comparisons.

Discussion
The major findings of the present study were that STZ induced diabetic rats showed significant weight loss, muscle incoordination, thermal and cold hyperalgesia, decreased grip strength, increased % GHb, electrophysiological abnormalities like decreased NCV and histological abnormalities when compared to normal rats. Treatment of diabetic rats with T4, BT and combination significantly improved the diabetes induced above deficits. Our results indicated that rats with diabetes induced by STZ showed body weight reduction during the experimental period. T4, BT and combination improved body weight from the initial value. Rats treated with combination exhibited less percentage of loss of body weight compared to T4 and BT alone thus the combination improved general health of rats by improving the body weight. DPN is associated with neuropathic pain which is most common in DPN, thus we evaluated the nociception in diabetic rats. Nociception was evaluated by thermal, cold hyperalgesia and was well evident in diabetic rats, which is in accordance with several other reports [39,37]. In the present study a significant reduction in nociception with T4, BT and combination treatment for five weeks was observed. The combination of T4, and BT exhibited synergistic effect on reducing nociception. The effectiveness of T4, BT and combination in neuropathic pain is further assessed by measurement of grip strength and muscle incoordination by grip strength meter and rotarod apparatus. Rotarod test was performed to examine the motor incoordination [40,38]. The Rotarod experiment demonstrated the impairment of the motor function and coordination in the diabetic rats. Diabetic rats showed shorter fall off time from the rotating rod when compared to control, suggesting impairment in their ability to integrate sensory inputs with appropriate motor commands to balance their posture. The T4, BT and combination treated diabetic rats increased the fall off time from the rotating rod compared to STZ-induced diabetic rats. Our results showed that T4, BT and combination normalize the motor function and coordination thus helps to maintain their posture during movement on the rod. The severity of diabetic neuropathy has been associated with decreased muscle strength in both type 1 and type 2 diabetes [41,39]. In our study we observed significant improvement in motor behavior particularly grip strength in addition to motor incordination after treatment with T4, BT and combination. The combination of T4, and BT thus exhibited synergistic effect on muscle incordination and gripstrength Marked increase inpercentage of Glycosylated haemoglobin (GHb) has been reported in previous studies in diabetic rats [42,40].
The levels of GHb is the marker of state of diabetes over a period of 90 days. Similar observations were found in diabetic control rats. In our study T4, BT and combination treated diabetic rats showed decreased HbA1levles. The decrease levels of HbA1 in T4 treated ratscould be due to decreasing elevated glucose by promoting cell differentiation into insulin-producing β-cells, upregulation of insulin, glucose transporter protein-2 transcripts, and insulin release [19], thus less glucose available for glycation with hemoglobin. The inhibition activity of T4 on glycation of proteins In Vitro was also measured in our study. T4 inhibited AGE formation In Vitro could be the contributing factor in inhibition of GHb in diabetic rats. Similar trend was observed in BT and combination treated rats. BT is a transketolase (TK) activator [43,41]. BT was shown to prevent experimental diabetic retinopathy and In Vitro hyperglycemia-induced endothelial dysfunction. The effects of benfotiamine on in vivo endothelial function remained unknown. BT has shown to inhibit hexosamine pathway, advanced glycation end product (AGE) formation pathway and the diacylglycerol (DAG) protein kinase C (PKC) pathways. BT was significantly decreased levels of HbA1 as discussed earlier could be due to inhibition of AGE formation as it was evidence by inhibition of In Vitro AGE formation in our study. The protective role of BT also probably due to its activity as co enzyme in various biological pathways [44,42]. The combination of BT and T4 has also shown synergistic effect in decreasing GHb.
A number of reports indicate that DPN is a hypoxic neuropathy. Decreased nerve blood flow may lead to decreased nerve conduction velocity (NCV) due to lower Na+-K+-exchanging ATPase activity [4]. Reactive oxygen species (ROS) such as superoxides and hydroxyl radicals cause vascular endothelial damage and reduced nitric oxide mediated vasodilatation. Studies have also showed evidence that superoxides and proximitized impairs endothelium dependent vascular relaxation of epineural arterioles of the sciatic nerve in diabetic rats [45,43]. In addition to vascular mechanisms nonvascular mechanisms like impairment of neurotrophic support have also been reported to cause nerve conduction deficits in DPN [46,44]. Enhancement of neurotrophic factors by prosaposinderived peptide was reported to preserve nerve conduction [47,45].The deficit in nerve conduction velocity was completely prevented by T4 treatment could be due to increased Na+-K+- exchanging ATPase activity, providing neurotrophic support and improving micro vascular circulation [48,46] further, T4 improved the endogenous antioxidants and decreased LPO in sciatic nerve homogenate. BT, combination treated rats were also exhibited augmented NCV could be due to the ability of BT to inhibit AGE formation, improved endogenous sciatic nerve antioxidant thus inhibiting AGE, free radicals induced nerve damage in our study. Thus these two vascular and nonvascular effects of BT and T4 would be the contributing factors for the synergistic activity in combination treated rats Morphological study of siatic nerve of normal rats showed closely packed nerve fibers normal endoneuria matrix separating the nerve fibers (Figure 14A), admixture of large and small diameter myelinated fibers and the thickness of the myelin sheath is proportionate the width of the axonal diameter (Figure 14 B). Conversly, diabetic rats displayed the histological damages like reduced nerve fiber density in the endoneurium, (loss of more of small myelinated fibers while large diameter fibers are better preserved), (endoneurial (Figure 15A), vascular thickening (diabetic microangiopathy) (Figure 15 B), However, myelin sheath was unaffected (Figure 14 B) by streptozotocin in DPN [49,47]. The altered sodium cell gradient due to impairment of Na+/K+ ATPase activity leading to altered membrane environment which in turn causes histological damages, and decrease myelin protein expression.
Figure 14: Histology of rat sciatic nerve of normal control group: (A) light microscopy transverse section showing closely packed nerve fibers and an occasional endoneurial blood vessel. (B) light microscopy transverse section showing individual nerve fibers and a central axon surrounded by a sheath of myelin.(C) longitudinal section showing the elongated schwann cell nuclei and longitudinally oriented axons with myelin sheaths.(D)transverse section of special stain for collagen highlights the endoneurial matrix separating the nerve fibers and collagenous component is stained blue.
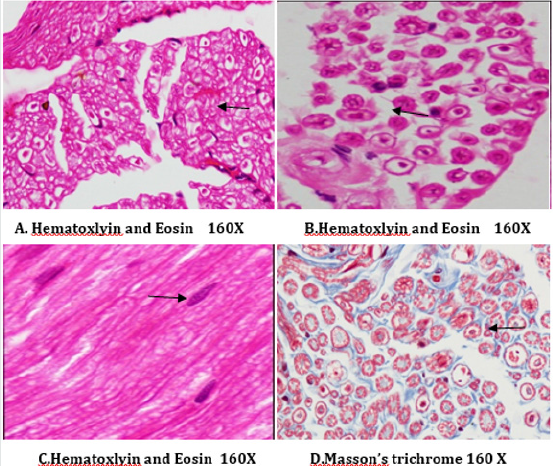
T4, BT and combination treated rats sections showed absence of diabetic vascular changes, no vascular thickening to mild vascular thickening (Figure 15B), normal density of fibers with preservation of small and large diameter fibers, presence of regenerating nerve clusters (Figures 16-18 ).Treatment with T4, BT and combination for five weeks almost completely prevented the histological damages induced by diabetes. T4, BT could probably prevent the histological damages induced by diabetes due to prevention of hyperglycemia induced vascular and non-vascular mechanisms.
Figure 15: Histology of rat sciatic nerve of STZ (Diabetes) control group. (A)light microscopy of transverse section showing reduced nerve fiber density in the endoneurium.(B) transverse section of special stain for myelin reveals more of small myelinated fibers affected while large diameter fibers are better preserved, myelin sheaths are of normal thickness.(C) transverse section show diabetic microangipathic changes with endoneurial vessel wall thickening and collagenous hyalinizationhighlighted by the masson’s trichrome stain (D).
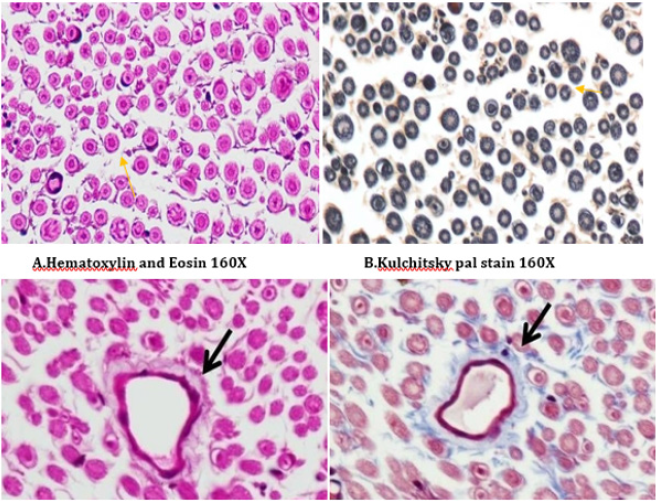
Figure 16: Histology of rat sciatic nerve of STZ (Diabetes) control treated with thyroxine. (A)light microscopy of transverse section showing preserved fiber density. (B) transverse section showing absence of diabetic vascular changes no vascular thickening to mild vascular thickening (arrow). (C) transverse section of special stain for myelin show near normal density of fibers with preservation of small and large diameter fibers, presence of regenerating nerve clusters.
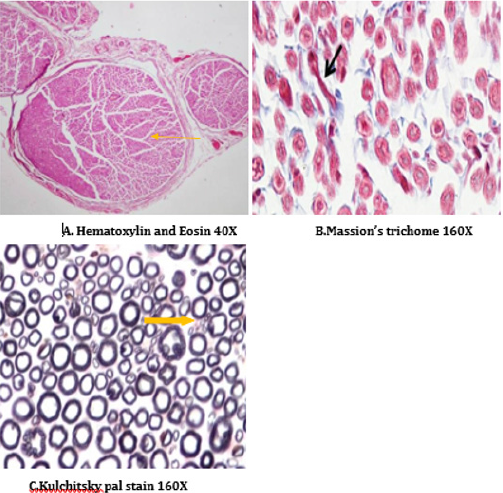
Figure 17: Histology of rat sciatic nerve of STZ (Diabetes) control treated with benfotiamine. (A)light microscopy of transverse section showing normal density of nerve fibers. (B) transverse section showing no vascular thickening.(C) transverse section of special stain for myelin show normal density of small and large diameter fibers, the myelin sheaths are stained dark.
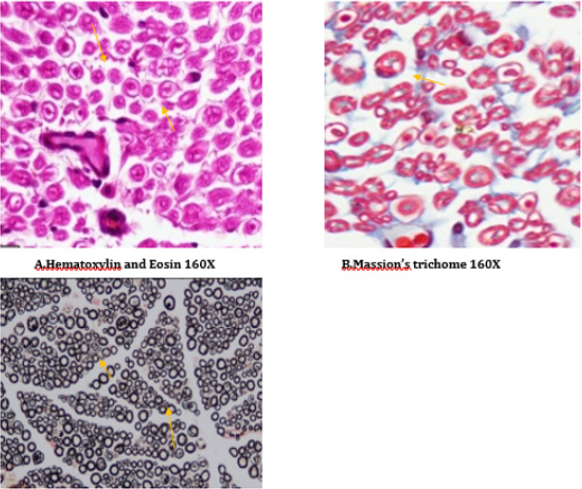
Figure 18: Histology of rat sciatic nerve of STZ (Diabetes) control treated with combination of thyroxine and benfotiamine. (A) light microscopy of transverse section showing sparse endoneurial interstitial inflammation in one case. (B) transverse section showing no endoneurial vascular thickening (C) transverse section of special stain for myelin show preserved density with presence of large and small diameter fibers.
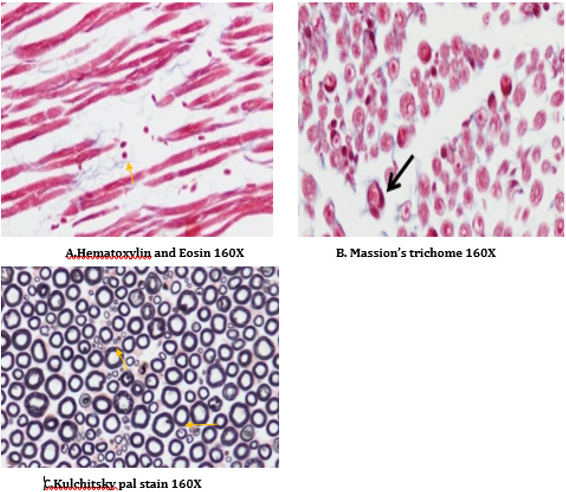
Conclusion
Treatment with T4, BT, and combination effectively prevented many of the behavioural, electrophysiological and histological manifestations of diabetes induced peripheral neuropathy by decreasing thermal and cold hyperalgesia, improving motor incoordination, grip strength, NCV, fiber density.
To Know More About Open Access Publishers Please Click on Lupine Publishers
Follow on Linkedin : https://www.linkedin.com/company/lupinepublishers
Follow on Twitter : https://twitter.com/lupine_online
Lupine Publishers| Semaglutide versus liraglutide for treatment of obesity
Lupine Publishers| Journal of Diabetes and Obesity Abstract Background: Once weekly (OW) semaglutide is a glucagon-like peptide...

-
Abstract Aims: To evaluate in a real practice setting effectiveness, safety and adherence to weekly subcutaneous semaglutide fo...
-
Today is the time to be thankful, remember good times, and embrace those who enrich our lives. I’m thankful for a lot of things. Happy Tha...
-
Lupine Publishers| Journal of Diabetes and Obesity Abstract Background: Once weekly (OW) semaglutide is a glucagon-like peptide...