Lupine Publishers| Journal of Diabetes and Obesity
Abstract
A recent study using next-generation RNA sequencing was reported on genome-wide changes in gene expressing in the skin between patients with type 2 diabetes mellitus, compared to non-diabetic patients. Ex-post review, based in part on both the existence of lipid droplets, peridroplet mitochondria and cytoplasmic mitochondria, selected in the gene metabolism category the most downregulated gene TPD52L3, and in the gene regulation category the most downregulated gene NKX2-1. There is strong evidence that these two genes are involved in the disease process of type 2 diabetes mellitus.
Keywords: Gene Expression; Lipid Droplets; Mitochondria; RNA Sequencing; Type 2 Diabetes Mellitus
Opinion
In an earlier study, it was proposed that the final consequence of
hereditary anomaly results in the development of type 2 diabetes,
which already emerges in the prediabetic phase. It was thought to
occur due to an increased flux, as compared to the healthy controls
where protons (H+-ions) from the mitochondrial intermembranespace
re-enter the matrix via uncoupling protein-1 (UCP1). This
causes hyperthermia in and around the mitochondria [1].
But the key question that remains to be answered here is for
the connection between the increased flow of protons and type 2
diabetes mellitus. In the past decade, a study reported on the visual
documentation of the possible interaction of lipid droplets with
mitochondria. This interaction was found to be quite intimate with
the involvement of membrane attached receptor proteins such as
SNAP23 [2]. Also, the cellular population of mitochondria in brown
adipocytes tissue could be divided into two subpopulations; i.e.
mitochondrial population having physical evidence of adherence
to a lipid droplet or peridroplet mitochondria, and a non-lipid
droplet-bound cytoplasmic mitochondrial population without any
adherence to lipid droplets [3,4].
Although both the peridroplet mitochondria and cytoplasmic
mitochondria are similar in their cell membrane composition they
differ in other fundamental respects [3,4]. A comparison of the
purified peridroplet mitochondria to cytoplasmic mitochondria
suggests that peridroplet mitochondria are more elongated,
whereas cytoplasmic mitochondria tend to be smaller. Also,
peridroplet mitochondria have enhanced oxidative phosphorylation
capacity, TCA cycle activity, ATP synthesis, as well as increased
ATP-dependent triglyceride synthesis compared to cytoplasmic
mitochondria. The measured fatty acid-driven respiration and UCP1
content in the isolated mitochondria suggests that for thermogenic
fat oxidation peridroplet mitochondria are not specialized
compared to cytoplasmic mitochondria [3]. This signifies that,
under healthy conditions, in the peridroplet mitochondria the
protons derived from free fatty acids (FFAs) and generated by the
electron-transport chain during the oxidation process of FA are
used for the production of ATP without any escape of protons via
UCP1 to produce heat. On the other hand, the protons generated
by the oxidation of cytoplasmic mitochondrial FA are mainly used
for the production of heat. So, peridroplet mitochondria have an increased coupled respiration, while cytoplasmic mitochondria
have an increased uncoupling activity. The existence of peridroplet
mitochondria demonstrates that the essential processes of fat
metabolism can be selectively confined to exclusive and segregated
subsets of mitochondria. The fatty acids intended for storage
undergo synthesis of triacylglycols followed by their storage in the
lipid droplets.
Most eukaryotic cells can store lipids in the form of droplets
[5]. Lipid droplets are cytosolic storage organelles at the center of
the lipid and energy homeostasis. They have a unique architecture
consisting of a hydrophobic core of neutral lipids, mostly
triacylglycerol and sterol esters and are enclosed by a phospholipid
monolayer membrane. This single layer is derived from the
endoplasmic reticulum, whereby triacylglycerols are synthesized
between the two leaflets of the endoplasmic reticulum membrane.
Associated with the monolayer is a specific set of proteins, which
decorates the surface of the lipid droplet but is absent from the
hydrophobic core [6]. These proteins associate with the membrane
through hydrophobic hairpins, amphipathic helices and fatty
acid modifications, and are also thought to control lipid droplet
positioning inside the cell and association with other organelles.
In 2016, researchers demonstrated that the exogenous
expression of human tumor protein D52 (TPD52) in the cultured
3T3 cells result in a significant increase in the numbers of lipid
droplets [7]. Starting with the bulging of a triglyceride lens within
the endoplasmic reticulum bilayer, lipid droplet biogenesis factors
including TPD52 are recruited to the lens structure and facilitate
the growth of the nascent lipid droplet [8,9]. Moreover TPD52-
expressing 3T3 cells form more lipid droplets following oleic acid
supplementation, which contributes to the lens formation [10]. As
a previous study has shown, an increase in carbon-carbon double
bonds in the acyl chains of phospholipids promotes the flexibility
of cellular membranes [11]. So, TPD52 expression increases lipid
storage, co-distributes with lipid droplets and is recruited to lipid
droplets to stabilize lipid droplets [12]. Moreover, it is interesting
to note that TPD52 knockdown decreased both lipid droplet sizes
and numbers [12].
Tumor protein D52 is the founding member of the TPD52-like
protein family representing four paralogous mammalian genes,
i.e. TPD52, TPD52L1, TPD52L2, and TPD52L3 [7,13,14]. The group
of Cao demonstrated that human TPD52L3 interacted with itself
and with TPD52, TPD52L1, and TPD52L2 [14]. The four human
linear proteins of this family are TPD52, TPD52L1, TPD52L2, and
TPD52L3, which consist of 184, 204, 206, and 140 amino acid
residues, respectively. Their first exon-coded protein located at
the N-terminal side is unique to each isoform, and all the members
contain a highly conserved coiled-coil motif located towards
the N-terminus which is required for homo- and heteromeric
interaction with other TPD52-like proteins [14,15] and share a
sequence identity of ~50% [7]. Byrne et al. proposed that TPD52
may exert and/or regulate its activities through interaction with
itself and its related proteins [15]. The coiled-coils were predicted
by use of pairwise residue correlations and were not based on their
crystal structures [16].
Figure 1: Alignment of the human TPD52 (upper row) and the human TPD52L3 (lower row) protein sequences. Amino acid residues are indicated by single letters. Vertical lines indicate identical residues and colons/dots indicate highly/weakly conserved residues.
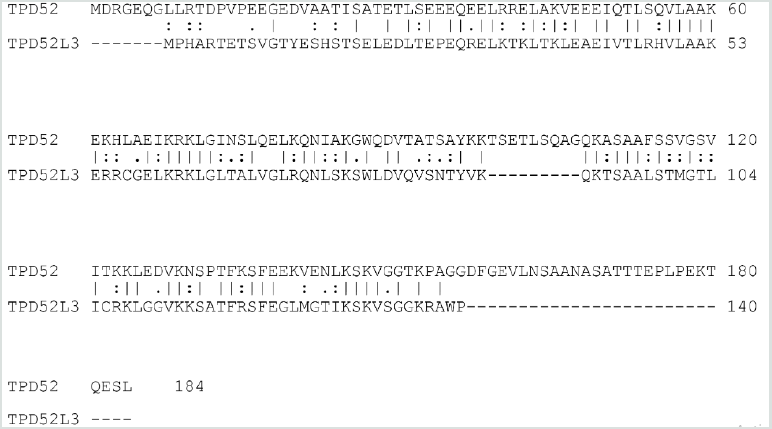
The analysis was focused on the sequences of TPD52 and TPD52L3. The two sequences revealed an obvious similarity, they share 63 identical positions and 42 similar positions resulting in an overall homology of 57.1% (Figure 1). The coiled-coil motif near the N-terminus was found in all TPD52-like proteins. Uniprot predicts a coiled domain for residues 28-57 of TPD52L3, and also for residues 21-70 of TPD52 [17]. The common part of these two coiled-coil protein sequences has an overall homology of 67.9% (Figure 1). The information regarding the amino acid sequences of human TPD52 (P55327-2) and TPD52L3 (Q96J77-1) was retrieved from the UniProtKB/Swiss-Prot databank. Interestingly, although the coiled-coil motif is very important for the interactions mediated by TPD52-like proteins [15,18], the TPD52L3 shortened coiled-coil motif successfully interacted with TPD52-like proteins.
A recent study using next-generation RNA sequencing was reported on genome-wide changes in gene expression in the skin between patients with type 2 diabetes mellitus, compared to nondiabetic patients [19]. The most downregulated gene of patients with type 2 diabetes in the gene metabolism category is TPD52L3 with a “log2 fold change” value of -28, compared to skin samples from non-diabetic patients. So far, this gene has not been linked to type 2 diabetes or wound healing.
The fact that the exogenous expression of human TPD52 increases the number of lipid droplets [7], TPD52 knockdown decreases the number of lipid droplets [12], and the activity of TPD52 depends on the interaction with TPD52L3 [14], support the idea that the major function of TPD52L3 is the lipid storage at the center of the lipid and energy homeostasis [8,9]. In other words, in brown adipocytes tissue, it seems likely that the significant downregulation of TPD52L3 causes a reduction in the number of lipid droplets in the skin samples of type 2 diabetes mellitus patients.
This indicates that an essential reduction in the lipid droplets suggests a substantial decrease in the peridroplet mitochondria for patients with type 2 diabetes and consequently an increase in the saturated plasma FFAs. As the unsaturation index (UI; number of carbon-carbon double bonds per 100 fatty acyl-chains) of FFAs from human white fat cells is substantially lower compared to the UI of serum FFAs in the healthy controls (85.5 and 191.9, respectively), these events force a shift from unsaturated to saturated acyl chains in the phospholipids of both the erythrocyte and vascular membranes [20]. This reduction in UI translates into an increase in the attractive forces between the mutual membrane phospholipid acyl chains, which redistributes the lateral pressure profile of the cell membrane [21]. This redistribution reduces the pore diameter of the transmembrane glucose transport channels of all Class I glucose transporter proteins, leading to a marked reduction in the transmembrane glucose transport [22].
On the other hand, the increased uncoupling activity of the cytoplasmic mitochondria [4] takes up the remaining fatty acid oxidation, including the formation of protons. The rationale is that the overall balance between the number of protons which re-enter the matrix through ATP synthase on the one hand, and the number of protons which re-enter the matrix through UCP1 on the other hand, might shift to the latter side, which in turn promotes an increase in the production of heat. To keep a narrow range of mitochondrial temperature compatible with life, the slow-down principle enters into force, which also results in an essential reduction in UI [1]. This chain of events is a blueprint of the development of type 2 diabetes mellitus.
A second result of the earlier mentioned genome-wide analysis study is the most downregulated gene in the gene regulation category, NKX2-1, of type 2 diabetes patients with a “log2 fold change” value of -28 compared to skin samples from non-diabetic patients [19]. Notably, a study also reported a novel heterozygous mutation in exon 3 of the NKX2-1 gene, which is related to a reduction in the muscle mitochondrial respiratory chain complex activity, a characteristic of type 2 diabetes [23]. It is to be noted that the reduced mitochondrial activity is one of the characteristics of type 2 diabetes [24]. This may be in advance of the patients with type 2 diabetes mellitus as the reduced mitochondrial activity implies a reduction in heat production.
Finally, it is worth considering about the potential benefit of the use of (modified) synthetic TPD52L3 for combating the adverse effects of type 2 diabetes mellitus.
Briefly, the idea is that two genes are pertinently involved in the disease process of type 2 diabetes mellitus: one concerns the downregulation of human TPD52L3 gene expression, which yields a significant reduction in the lipid droplets, whereas the second one relates to the downregulation of human NKX2-1 gene expression which reduces the mitochondrial respiratory chain activity (Figure 2).
Figure 2: Although the results of genome-wide screen for type 2 diabetes susceptibility genes are still under debate, a refined working hypothesis proposes that the primary effect of the downregulation of the human genes TPD52L3 and NKX2-1 generates an increased flux of mitochondrial intermembrane-space protons through UCP1 into the matrix, which causes an increase of extra heat. This process initiates the slow-down principle. UCP: Uncoupling protein; FFA: Free fatty acid; GLUT: Glucose transporter.
Read More Lupine Publishers Diabetes and Obesity Journal Articles: https://diabetes-obesity-lupine-publishers.blogspot.com/